GLASGOW MITOCHONDRIA CENTRE
We are a collective of researchers at the University of Glasgow who xxxxxxxxxxxxxxxxxxxxxxxxxxxxxxxxxxxxxxxxxxxxxxxxxxxx
Research Laboratory Groups
Dr Payam Gammage, School of Cancer Sciences
Professor Richard Hartley, School of Chemistry
Professor Vignir Helgason, School of Cancer Sciences
Dr Thomas MacVicar, School of Cancer Sciences
Dr John Mercer, School of Cardiovascular & Metabolic Health
Professor Neil Metcalfe, School of Biodiversity, One Health & Veterinary Medicine
Professor Alberto Sanz Montero, School of Molecular Biosciences
Professor Colin Selman, School of Biodiversity, One Health & Veterinary Medicine
Professor Lilach Sheiner, School of Infection & Immunity
Professor Stephen Tait, School of Cancer Sciences
Professor Helen Walden, School of Molecular Biosciences
Dr Lorraine Work, School of Cardiovascular & Metabolic Health
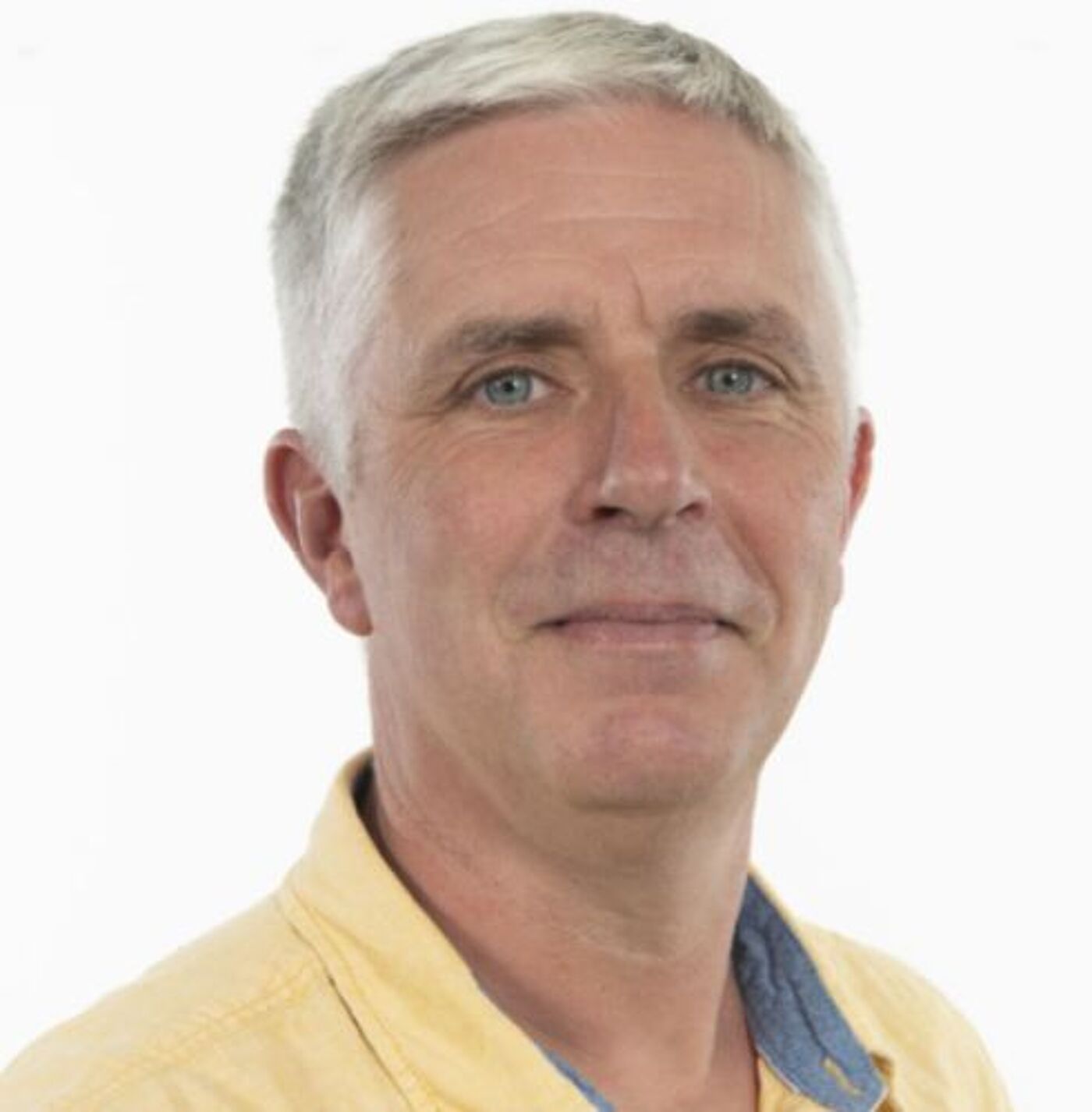
Tait Group
Stephen Tait’s lab investigates how mitochondria regulate cell death and inflammation, with the goal of targeting these processes to improve cancer treatment. Specifically, the Tait lab has found that non-lethal apoptotic stress can be oncogenic, through limited mitochondrial permeabilisation (Ichim et al, Mol Cell, 2015; Cao, et al, Dev Cell, 2022). Secondly they found that killing cells under caspase inhibition can be immunogenic, positioning caspase inhibitors as potential anti-cancer therapies (Giampazolias et al, Nat Cell Biol 2017). This has spurned an interest in understanding how mitochondria regulate immunogenicity of cell death, leading to the discovery that mitochondrial permeabilisation causes mtDNA release leading to cGAS-STING activation (Riley et al, EMBO J, 2018). Finally, his lab is investigating crosstalk between senescence and apoptosis in the context of mitochondrial regulated inflammation (Victorelli et al, Nature 2023). The Tait lab ethos is centred on discovery based science using a variety of cell biology approaches coupled with in vivo modelling.
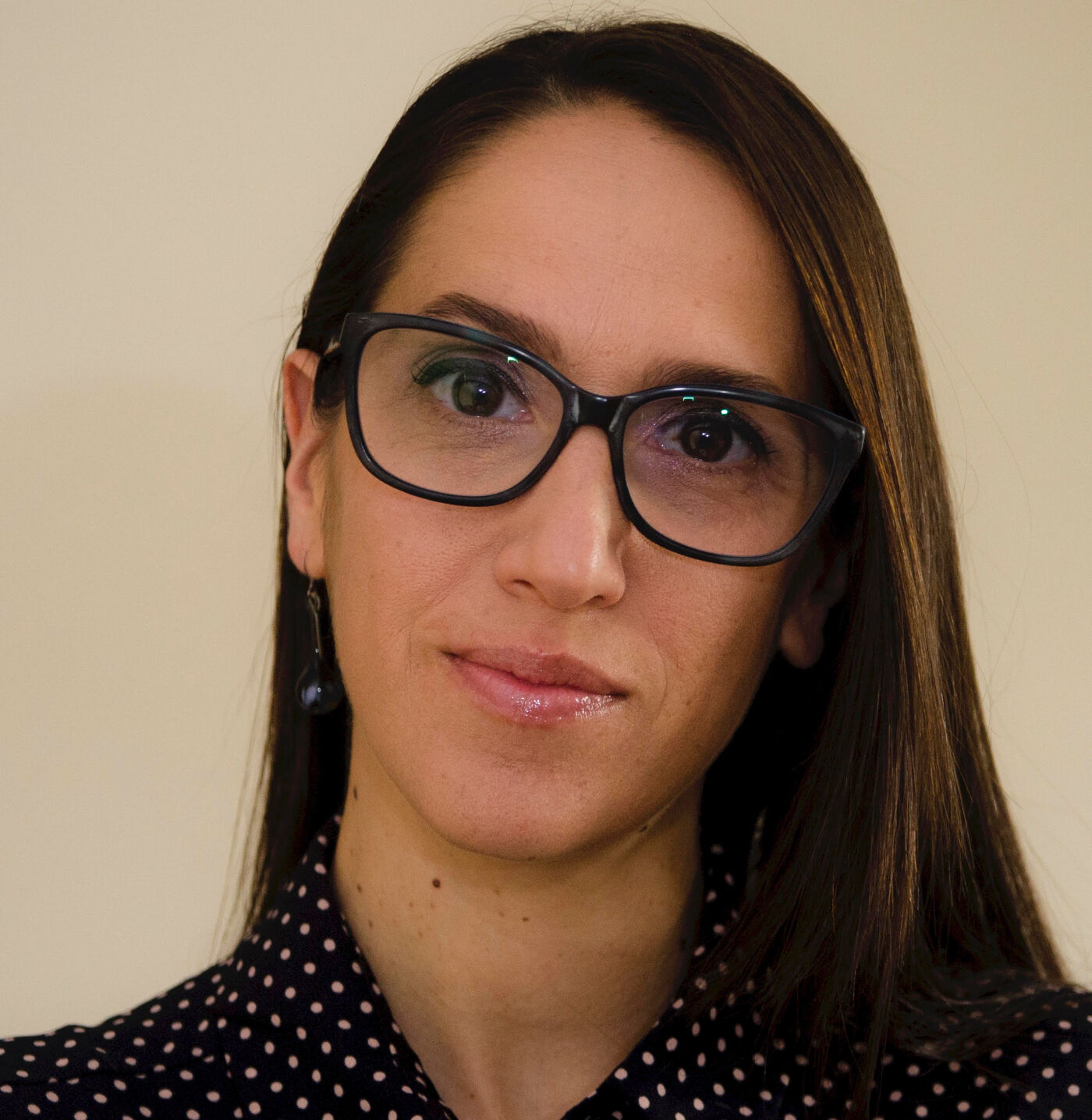
Sheiner Group
Our understanding of mitochondrial biology relies primarily on a handful of model organisms such as mammalians and yeast, but the study of divergent organisms has started revealing novel and unknown mitochondrial features and functions. My team contributes to this important shift of focus through the study of the mitochondrion of Toxoplasma gondii, a model for an understudied lineage that is divergent from yeast and mammals.
Since Toxoplasma is a pathogen, our discoveries sometimes also pinpoint new parasite-specific structures and feature that are potential targets for intervention.
Key techniques: Parasite genetic manipulation and cell biology. Microscopy. Mitochondrial biochemistry and structural biology.
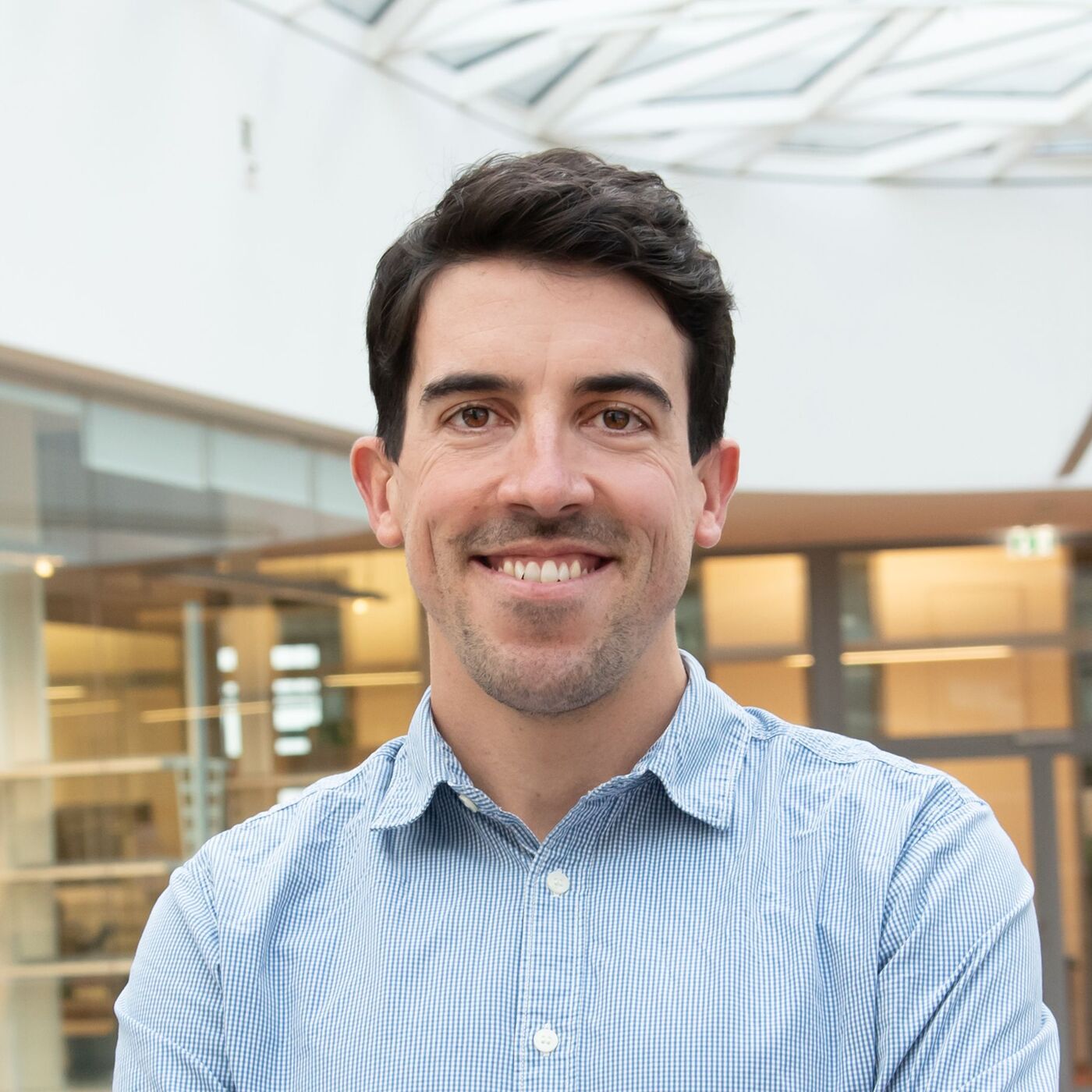
MacVicar Group
I established my research group at the CRUK Beatson Institute in December 2021 with the overarching aim to uncover the metabolic vulnerabilities of cancer by targeting mitochondrial metabolite transporters and mitochondrial nucleotide metabolism. We combine cell biology approaches, including genetic screening and high-content imaging, with 3D tumour models to dissect the mechanisms of mitochondrial reprogramming during cancer progression. We also have expertise in methods to study mitochondrial quality control and compartmentalised metabolism.
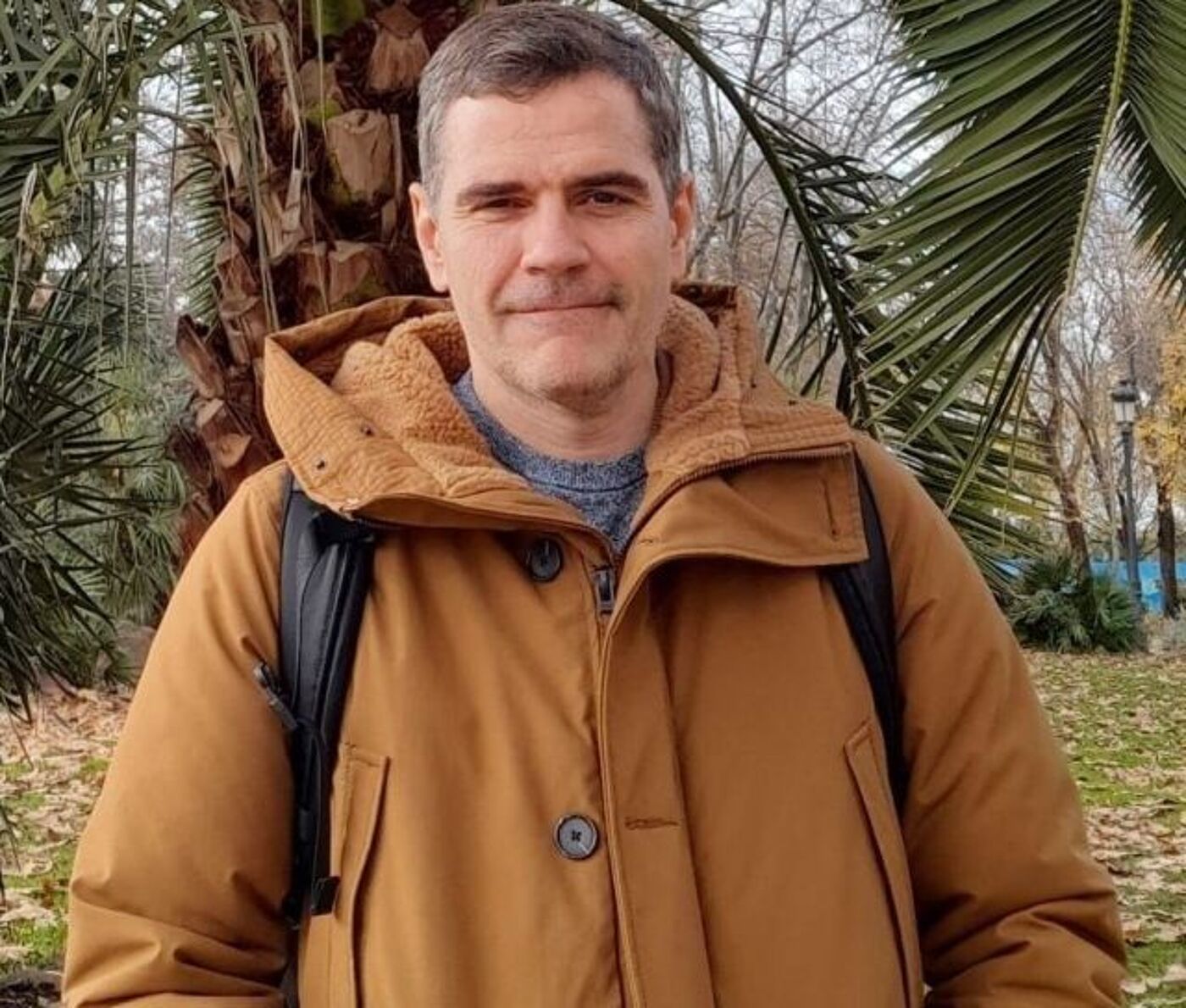
Sanz Montero Group
At Sanz's lab, we use fruit flies to understand how and why we age. Our focus is on mitochondria and their influence on lifespan. We're developing models for reprogramming metabolism in older individuals with the goal of restoring youthfulness. By leveraging state-of-the-art molecular biology and big data analysis, we're charting new paths in ageing research to help people live healthier for longer.
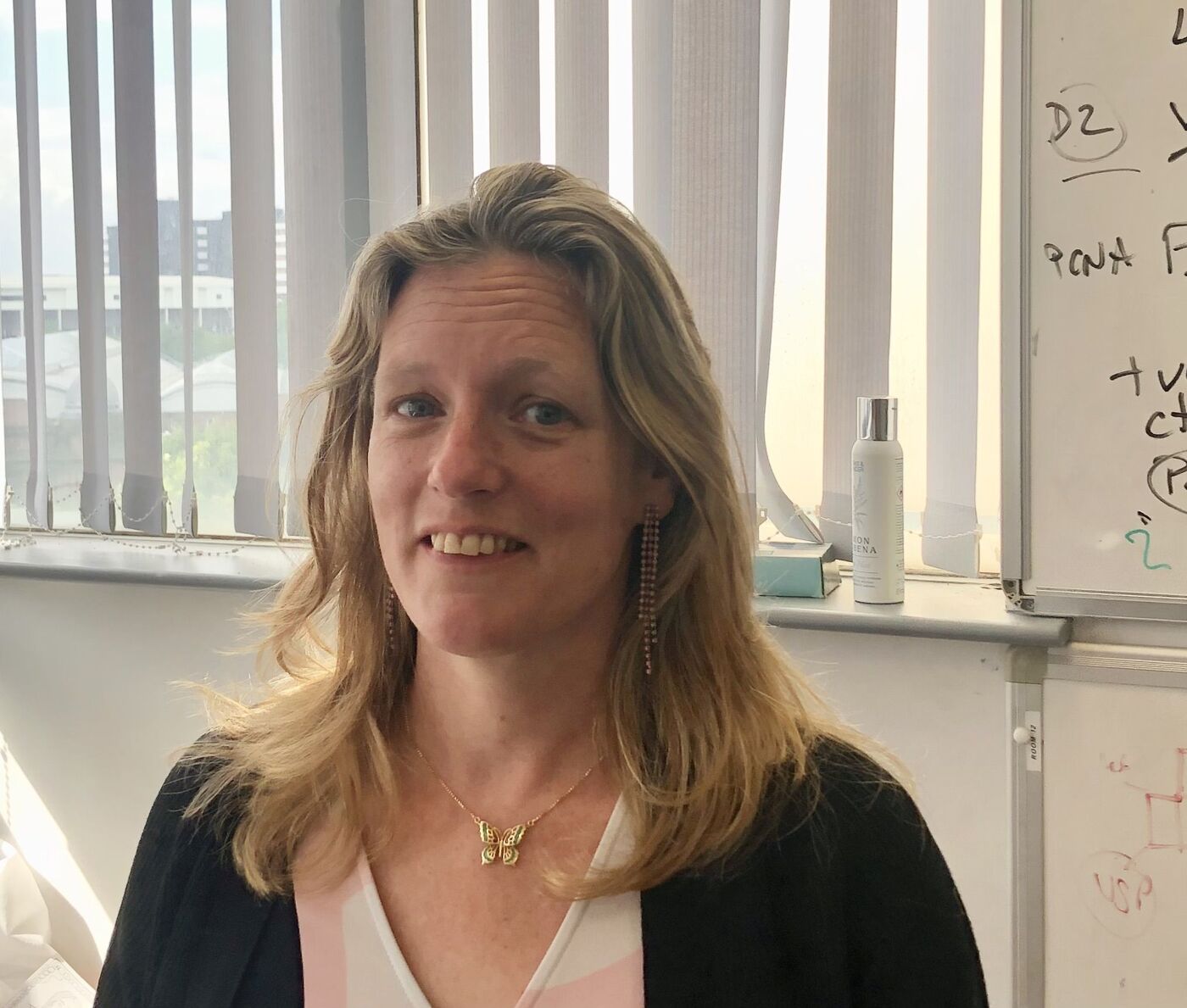
Walden Group
Our research focuses on understanding the molecular mechanisms underlying protein ubiquitination.
Ubiquitination of a target protein is an essential cellular regulatory signal, found in all aspects of cell biology including DNA replication and repair, the immune response and endocytosis. Consequently, dysfunction in the ubiquitin system is implicated in many disease states including neurodegeneration and cancer. Although many aspects of the mechanisms of ubiquitination are well understood, current open questions in the field include how the type of ubiquitination event is selected (eg mono- versus polyubiquitination, polyubiquitin linkage or branched chain type, multimono-ubiquitination), and how the substrate itself is targeted by the conjugation machinery.
We are exploiting 2 model, medically relevant systems to answer these questions. First, the Ring-InBetweenRing-Ring ligase, Parkin, is a promiscuous ubiquitin-ligase with many putative substrates, capability for forming different chain types and lengths, and interactions with different E2s. Mutations in Parkin cause recessive inherited Parkinsonism, and Parkin is also a tumour suppressor. It also plays a role in mitophagy. We use this model to understand tolerance and promiscuity in E3s. Second, the Fanconi Anaemia DNA repair pathway contains one E3 ligase unit (FANCL), responsible for catalysing solely mono-ubiquitination of 2 well-defined substrates. We use this model to understand specificity within the ubiquitin system. We are taking a combined structural and biochemical approach to understand how these E3s interact with their binding partners and targets, and how they might be regulated.
Techniques we use are structural biology (X-ray crystallography, and cryo electron microscopy), biophysical approaches, and biochemistry.
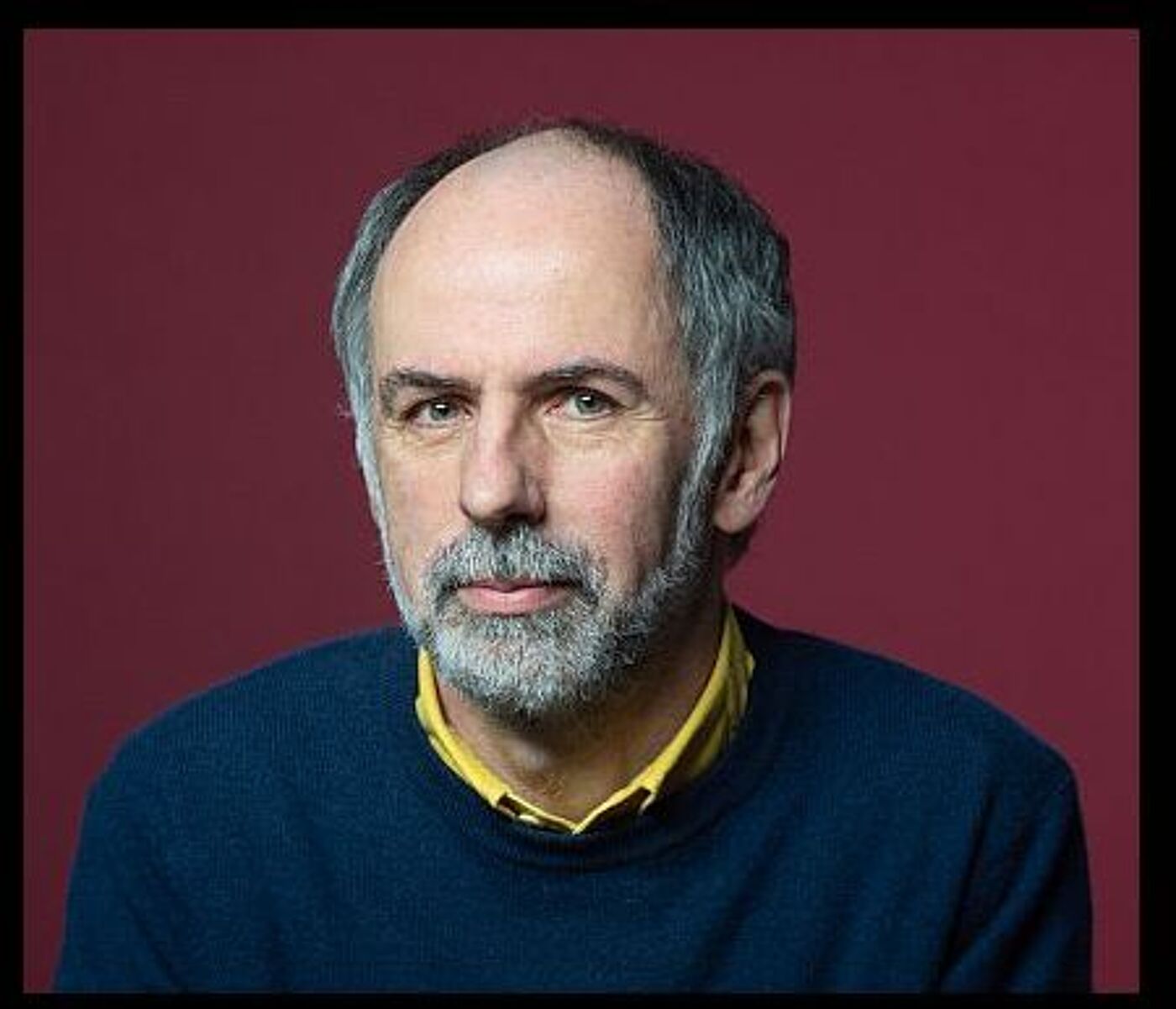
Metcalfe Group
Neil Metcalfe's research group and collaborators focus on the causes and consequences for performance of intraspecific variation in mitochondrial function. Why do some individuals have mitochondria that are twice as efficient as others at converting substrates and oxygen into ATP? Does this greater efficiency allow them to function at a higher level, and does it come at a cost, for instance of greater oxidative damage? Are there ecological consequences of variation in mitochondrial efficiency? These issues are explored in a range of vertebrate species, particularly fish.
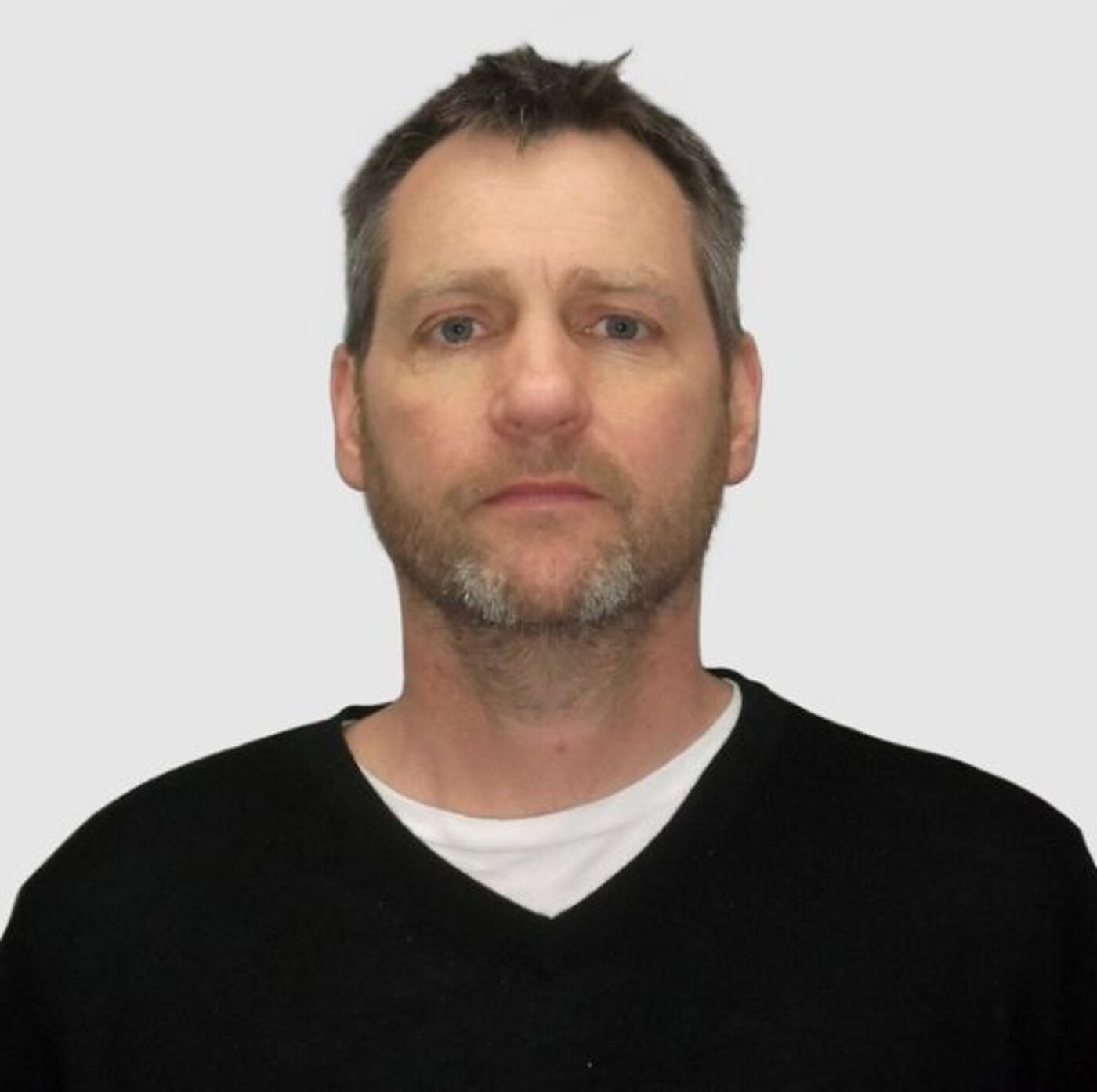
Selman Group
My research aims to understand conserved mechanisms underlying the ageing process. To this end I have significant experience in using dietary, genetic, and pharmacological interventions to modulate lifespan and health in mice, most notably interventions that extend these (e.g. insulin/IGF-1 and mTOR pathways, dietary restriction). In addition, I have expertise in studying ageing and metabolism in non-model organisms (laboratory and field conditions) and using in vitro approaches. My group utilises a number of approaches including whole-animal and mitochondrial metabolism, mouse lifespan, healthspan (frailty, rotarod, grip strength, glucose homeostasis, fecundity), RNAseq, metabolomics, histology and molecular biology techniques.
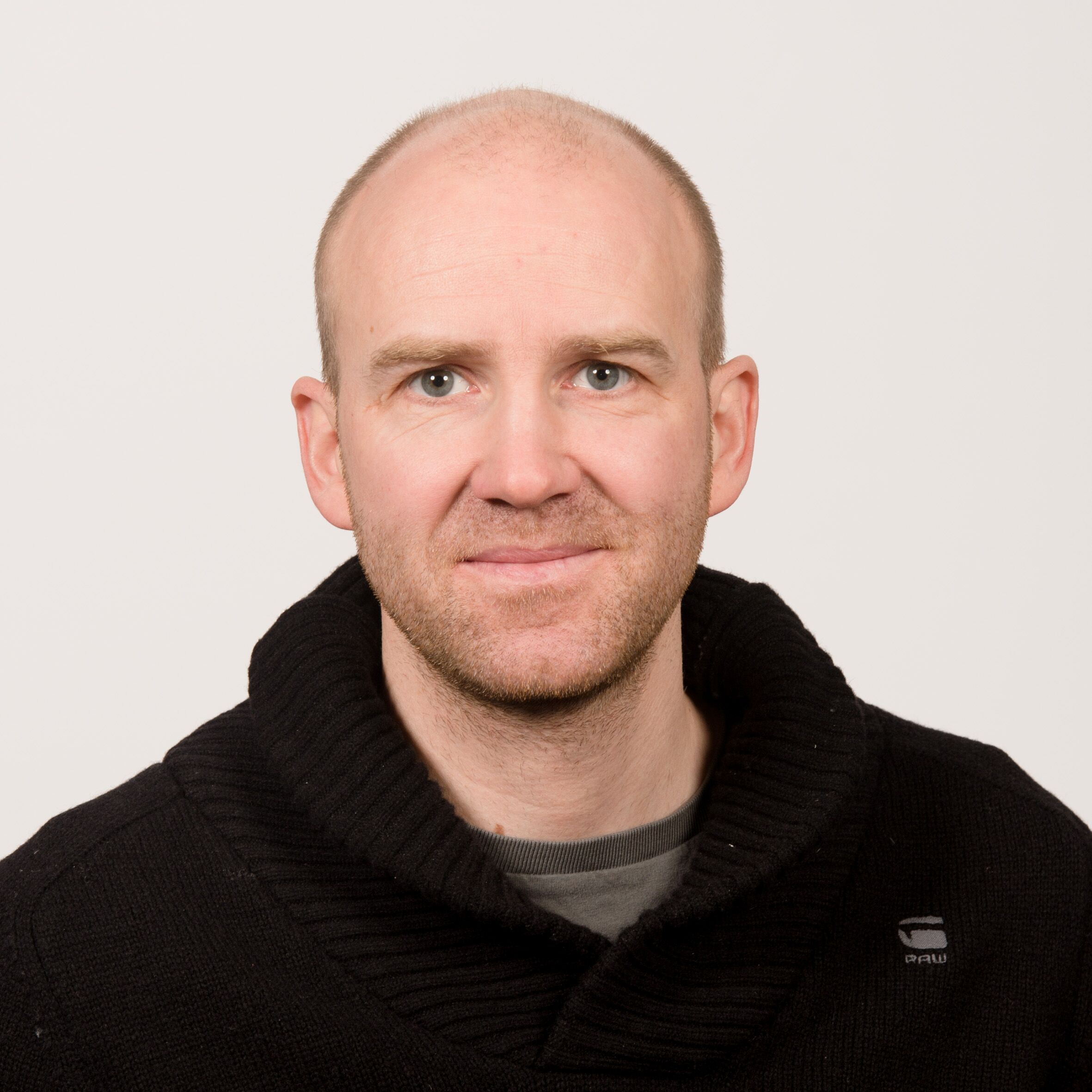
Helgason Group
Overarching aim of our work is to identify targetable drug resistance mechanisms in myeloid leukaemias. Our lab demonstrated that chronic myeloid leukaemia stem cells have increased mitochondrial content and activity when compared with normal blood cells. Therefore, we are focusing on further investigating mitochondria regulation and function, in an attempt to targeting appropriate therapy to the stem cell compartment to remove the population in which drug resistance occurs. We routinely use Seahorse Analyzer to measure mitochondrial respiration, both in cell lines and patient derived cells, under normoxic and hypoxic culture conditions. We have also generated several leukaemia cell line models to measure autophagy-mediated mitochondrial degradation (mitophagy) using flow cytometry or microscopy.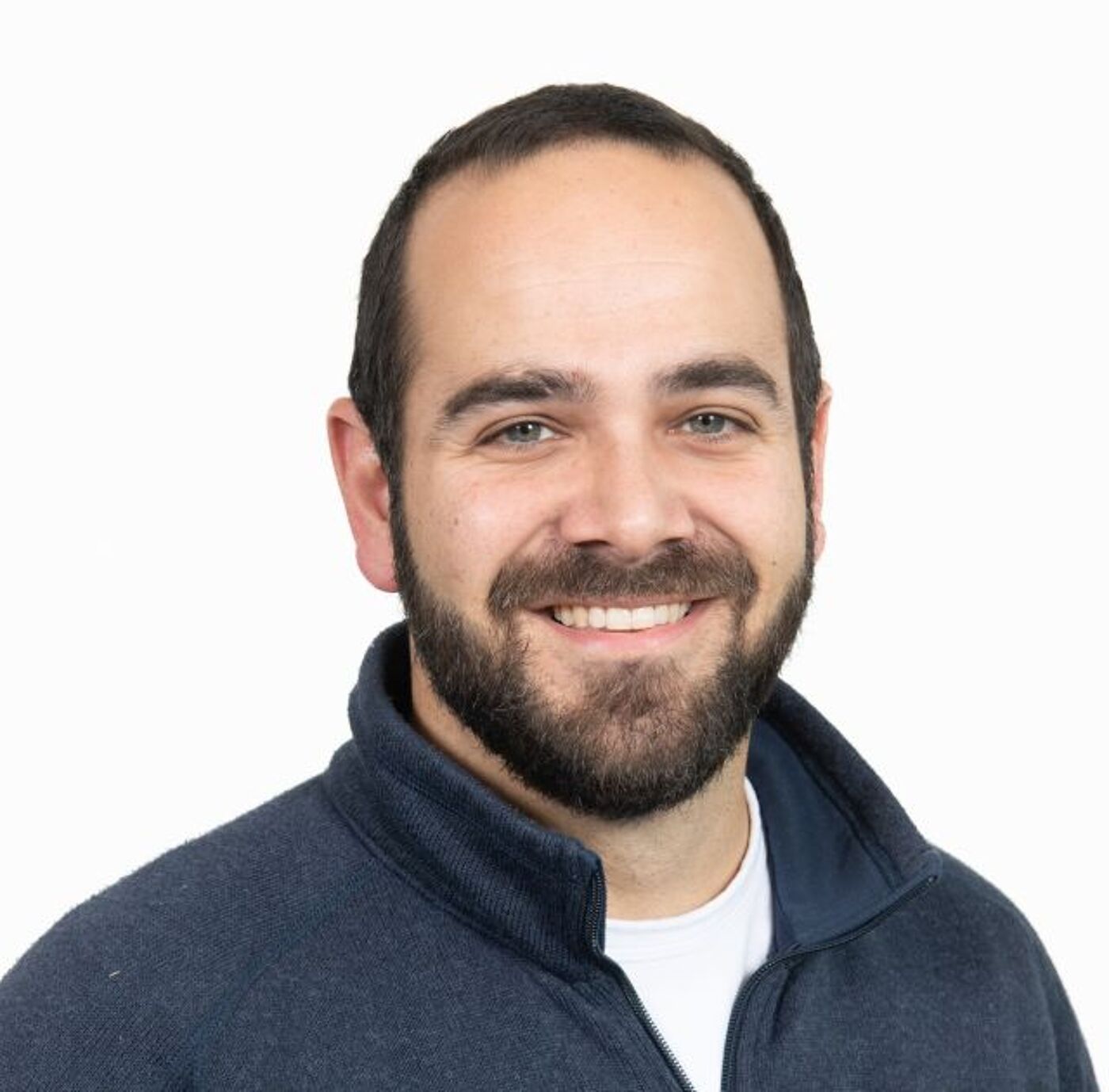
Gammage Group
Mitochondrial Oncogenetics Laboratory, CRUK Beatson Institute
Cancer, in its many forms, is substantially metabolically diverged from healthy tissue. It is now emerging that a major source of this metabolic divergence is mutation of mitochondrial DNA (mtDNA).
Mutation of mtDNA causes metabolic disease in rare hereditary mitochondrial disorders, due to primary dysfunction of the mitochondrial respiratory chain. Efforts from our group and others have determined that mutation of mtDNA is one of the most common mutational events in the cancer genome, present in up to 60% of all tumours. However, due to lack of tools and capacity to interrogate function, this convergence of cancer genetics and metabolism has remained under-investigated. Our lab combines expertise in mitochondrial biology, genetics, genomics and genome engineering with in vitro and in vivo modelling of cancer to address questions of cancer metabolism, tumour biology and therapeutic vulnerability.
Key expertise & technologies:
- Mitochondrial genomics – NGS, pyrosequencing, single cell approaches
- Mitochondrial genome engineering – nucleases, base editors
- Metabolism – mitochondrial function (OCR/ECAR, multi-wavelength redox spectroscopy), GC/LC-MS metabolomics, isotope tracing/flux analysis
- Cancer biology – organoids, cell migration/invasion/proliferation, xenograft/syngeneic grafts, transgenic/GEMM models of mtDNA mutation and cancer, flow cytometry, histology, scRNAseq, chemotherapy/immunotherapy
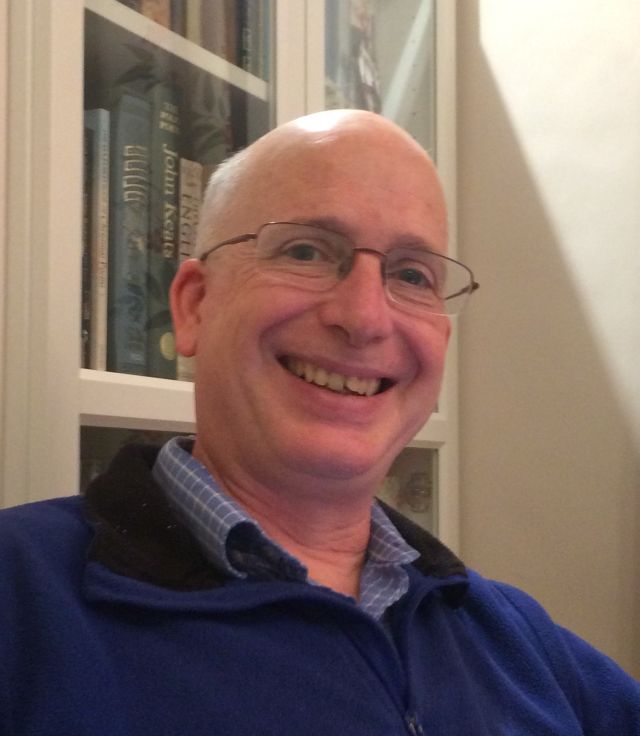